The 6th (2006) Yamazaki-Teiichi Prize Winner Measurement Science and Technology
Electrical Detection of Biomolecular Recognition Using Genetic Field Effect Transistor and its Application to Genetic Analyses
Winner | ||
---|---|---|
Yuji Miyahara | ||
History | ||
Mar. 1985 | Completed Doctoral Course at Dept. of Electrical and Electronics Engineering, School of Science and Engineering, Tokyo Institute of Technology(Doctor of Engineering) | |
Apr. 1985 | Joined Central Research Laboratory, Hitachi Ltd. | |
Apr. 1988 | Visiting Researcher, Swiss Federal Institute of Technology(ETH) | |
Oct. 2002 | Director, Bioelectronics Group, Biomaterials Center, National Institute for Materials Science | |
Apr. 2006 | Also undertook position of Professor at Dept. of Materials Engineering, School of Engineering, Graduate School of The University of Tokyo | |
Present |
Winner | ||
---|---|---|
Toshiya Sakata | ||
History | ||
Mar. 2003 | Completed Doctoral Course at Department of Materials Science and Engineering, Graduate School of Engineering, Osaka University (Doctor of Engineering) | |
Apr. 2003 | Biomaterials Center, National Institute for Materials Science | |
Sep. 2006 | Dept. of Materials Engineering, School of Engineering, The University of Tokyo, Graduate School & Center for NanoBio Integration | |
Present |
Reason for award
For over 20 years, Yuji Miyahara has been consistently devoted to sensor research and development, through which he has created various innovative measurement methodologies. Deserving special mention is his development, together with Dr. A. Manz, of the world's first micro total analysis system (μTAS), the monumental treatise for which was released in 1990. Semiconductor microfabrication technology was applied to actualize an ultracompact liquid chromotograph, the actual performance and characteristics of which were presented. The concept of micromeasurement systems or microchemical reaction systems based on microfabrication technology became widely received thereafter. In November of this year, The 10th International Conference on Miniaturized Systems for Chemistry and Life Sciences (μTAS2006) will be held in Japan.
In progress made together with Mr. Sakata, Mr. Miyahara has advanced experiments that combine the principles of semiconductor elements with chemical reactions in solution. They applied this to the detection of biological molecules to further develop the functional analysis of DNA. In particular, the method designed to produce a variety of biomolecular recognition responses on FET gates yielded a new measurement methodology. Using the fact that DNA has a negative charge and that FETs are sensitive to changes in charge density on the gate insulation layer interface, they created sites for specific molecular recognition responses of DNA in the gate surface nanoregion. This has enabled the functional information for DNA molecules to be detected by means of electrostatic interaction with semiconductor electrons. In fact, experiments proved the basic principles of single-base polymorphism analysis and DNA base sequence analysis.
Although the first attempt to immerse FETs in aqueous solution was made long ago (1970), it was difficult to apply it to the detection of antigen-antibody reaction. For example, although the expanding size of antibodies is on the order of 10nm, their Debye length in physiological salt solutions is about 1nm. In keeping, antigens in solution bind with antibodies outside the electrical double layer, and the antigens' electrical charge will be shielded by antagonist ions. However, when a diluted buffer solution is used, Debye length is increased by one digit. In addition, Mr. Miyahara et al. noticed that because specific affinity with complementary DNA is shown even with short fragments of DNA on the order of 20 base lengths (6.8nm), hybridization (complementary strand synthesis) and other molecular recognition responses can be made within the limit of the Debye length. In addition, because of the strong binding force of DNA complementary strand binding, separation of the reaction process and the measurement process fortunately enabled measurement conditions to be closely controlled at the time of measurement.
The aforementioned biotransistor is manufactured using microfabrication technology. As the entire process from biomolecular recognition to electronic signal conversion is integrated within one chip, it is optimal for making entire systems compact. Thus, portable and compact biomolecular analysis systems can be actualized in kind. The advanced genetic testing conventionally done by large hospitals alone could now be done by clinics, hospital wards, satellite labs and other small-scale facilities. Additionally, the possibilities for application to drug discovery and genome research are considered to be major.
Background of research and development
Since 1970, when the first ion sensor using a field effect transistor (FET) was reported, active research has been conducted on ion sensors and biosensors combined with polymer membranes and immobilized enzyme membranes. However, the opinion that it is difficult to detect proteins, nucleic acids and other biomolecules of high molecular weight on FET gates was widely accepted. At the same time, DNA sequencing technology combining electrophoresis and fluorescence detection is widely applied in the field of gene function analysis, contributing substantially to the completion of the Human Genome Project (HGP). Recently, the need for DNA base sequence analysis for other living organisms has increased, making the development of convenient, high-throughput DNA base sequence analysis technology to replace the conventional DNA sequencer desirable.
Achievements
The prizewinners considered the issues with the conventional FET biosensors primarily in terms of the points specified below and, through the technical resolution of them, led the world in developing gene analysis technology employing a method to directly detect molecular charge. Integrating biomolecular recognition and the FET, the biotransistor is a new biodevice designed to recognize various molecular reactions on gates and to detect their specific binding. Using the negative charge that DNA molecules have, space for DNA molecular recognition reaction is created in the nanoregion of the gate surface and detection done through the electrostatic interaction of DNA molecular function information and semiconductor electrons. First, the prizewinners considered the (i) solution and (ii) control of the electrical double layer width (Debye length) of the gate insulation layer surface to be important for highly sensitive detection of DNA molecules on the gate surface. Thus, they optimized the base length for the DNA probe and the concentration of the buffer solution. Doing so made it possible to induce changes in molecular density within the limits of the Debye length. Second, owing to the substantial binding strength for DNA complementary strand binding, different from the conventional FET biosensors, they focused on separating the molecular recognition reaction and the charge measurement process. Being able to measure DNA charge in an environment with buffer solution concentration and other factors controlled made it possible to control the Debye length, as well. Third, not only conducting hybridization on the gate but molecular recognition reactions that DNA engages in, such as intercalation and elongation reaction, improved reaction specificity, actualizing high signal-to-noise (S/N) measurement. In particular, by combining elongation reaction using the enzyme Taq DNA polymerase with charge measurement, the ability to detect differences in single bases was confirmed, and single-base polymorphism analysis and DNA sequencing analysis were realized. With this method, DNA base sequences can be analyzed using one type of enzyme only, thus simplifying the reaction and measurement systems. As biotransistors can be manufactured with semiconductor microfabrication technology, integration and density growth are possible, making this a key technology for high-throughput DNA sequencing.
Meaning of the achievements
Gene analysis technology using biotransistor is based on a new principle: direct detection of molecular charge. Unlike with conventional fluorescence detection, lasers and optical systems are not necessary, making the technology effective for realizing compact gene analysis systems. In keeping, it is expected to serve in the provision of high-grade medical care at medium- and small-size hospitals, clinics and other accessible medical institutions. It is also expected that with the biotransistor, a device that specifically integrates the fields of electronics and biotechnology, research on the boundaries between different fields and in the areas in which they fuse will continue to advance.
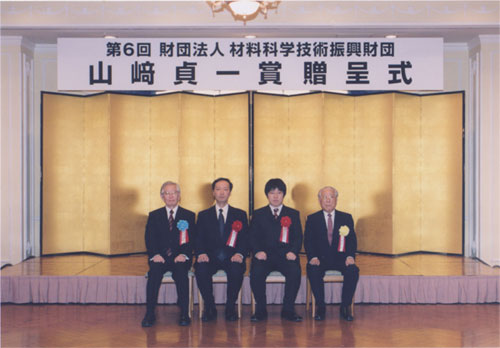